Podcast: Play in new window | Download
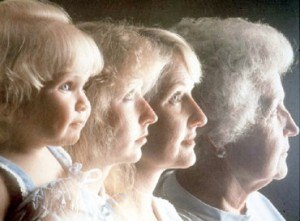
Continuing our examination of the biological mechanics of heredity.
Once again, this material is dense, so to fully appreciate everything that’s covered you may find it necessary to listen more than once. You might also like to consult the web page for this program to find the proper spelling for some technical terms, and for links to more in-depth explanations.
The last time we covered the macromolecular biosynthesis which goes on within every living cell – how proteins called polymerases transcribe DNA into RNA, and how proteins called ribosomes translate RNA into all the many proteins required for life to go on. We also touched briefly on the three main “domains” of life and the two main kinds of cells – the single-celled or unicellular prokaryotes, Archaea and Bacteria, and the unicellular and multicellular eukaryotes, or Eukaryota. It is this last class of life, the multicellular eukaryotic organisms, which includes all the forms of plant and animal life most familiar and most closely related to humankind, and which I therefore assign the most importance for the purpose of this primer.
This time we’ll review some key distinctions at the cellular level having to do with reproduction and genetic inheritance.
We’ve gotten a bit ahead of ourselves by talking about different types of cells before we even described what cells are. So we’ll start this time with the cell:
The cell (from Latin cella, meaning “small room”) is the basic structural, functional, and biological unit of all known living organisms. Cells are the smallest unit of life that can replicate independently, and are often called the “building blocks of life”. The study of cells is called cell biology.
Cells are small, in most cases too small to see with the naked eye. It was the invention of the microscope which made the ubiquity of cells and the significance of their workings visible.
The cell was discovered by Robert Hooke in 1665. The cell theory, first developed in 1839 by Matthias Jakob Schleiden and Theodor Schwann, states that all organisms are composed of one or more cells, that all cells come from preexisting cells, that vital functions of an organism occur within cells, and that all cells contain the hereditary information necessary for regulating cell functions and for transmitting information to the next generation of cells.
In multicellular forms of life there are many different types of cells, each specialized to perform one specific function or another – blood cells, muscle cells, and nerve cells, for example. In animals, the most critical distinction in specialization is between germ and soma. This distinction was first made by the German evolutionary biologist August Weismann in the 1880s.
According to Weismann’s germ plasm theory, inheritance only takes place by means of the germ line, or germ cells, also known as gametes, such as egg cells and sperm cells. All the other cells of the body, called soma or somatic cells, do not function as agents of heredity.
Weismann pointed out that a germline cell:
is immortal in the sense that it is part of a lineage that has reproduced indefinitely since the beginning of life and, barring accident could continue doing so indefinitely.
Soma cells, in contrast, are mortal and serve merely as a vehicle for the germ line. Together they both serve as a vehicle for the genetic information of the organism, its biological inheritance, its genes, which we know now is encoded in its DNA.
As we saw with biosynthesis, as a general rule the flow of genetic information goes only one way, from DNA to RNA to protein. And likewise, as we see here, for multi-cellular reproduction there is a similar one-way relationship between germ and soma. Though all cells contain and make use of DNA to do what they do, it is only the germ cells – egg and sperm – which play the key role of carrying the genetic information, the blueprints of the organism, from one generation to the next during reproduction.
Those DNA blueprints, carried by the germ cells, contain the information how to build all of an organism’s cells, both germ and soma. The germ cells carry the essence of life, whereas the soma cells are the workers and materials, just as within each cell the various proteins and carbohydrates which are constructed and can perform construction only according to the information encoded and contained in the nucleic acids.
Within cells, at the molecular level, this general one-way rule was first identified by Francis Crick, one of the two men credited with discovering the double-helix structure of DNA. He called this one-way relationship the central dogma of molecular biology.
The analogous general one-way rule at the cell level was identified decades earlier, by Weismann. That one-way relationship, whereby genetic information only passes to the next generation via the germ cells, not the somatic cells, is called the Weismann barrier. The idea of the Weismann barrier is central to what biologists today call the modern evolutionary synthesis, though they don’t express it in the same terms.
These general one-way rules are important for two reasons. They help explain how evolution works, and how it doesn’t work.
Can a mutation in the DNA of one of your liver cells be passed on to your children? No. Your liver cells, like most of the cells in your body, are soma, and therefore the DNA in them is not passed on to your children. Only mutations in the DNA within germ cells have any chance of being passed on.
This is why Lamarckism is wrong, at least for multicellular organisms, including humans. Lamarckism is the idea that an organism can pass on to its offspring characteristics that it acquired during its lifetime, also known as heritability of acquired characteristics or soft inheritance. I direct you to Race and Genetics – Part 3 and the next few podcasts after it for a previous discussion of Larmarckism and how it fits into the hijacking of race science by “anti-racist” jews.
I use the qualifier “general” when describing these one-way rules because there are some exceptions. If you read what microbiologists have to say about the Weismann barrier today, for example, you see they have discovered evidence of horizontal gene transfer, the exchange of genetic information between organisms outside the usual, more thoroughly understood reproductive mechanisms. Different species, especially unicellular forms of life, and especially prokaryotes, appear to be swapping genes through the activities of retroviruses. Retroviruses are able to transfer genes between species because they reproduce by integrating their code into the genome of the host. If the cell they infect is a germline cell then that integrated DNA can become part of the gene pool of that species.
That’s close to the ultimate in virulence – a parasitic lifeform screwing with the DNA of the organisms which host it, mixing them together. Imagine the damage retroviruses could do if they were to organize to manipulate cell-level media and politics in order to actively suppress any anti-retrovirus immune response from their hosts.
There is another pair of biological terms whose meaning seems very similar to germ and soma, and which today are in even more common use. The terms are genotype and phenotype. In my layman’s understanding, I’ve always regarded the meaning of genotype as roughly corresponding with germ, and phenotype with soma – genotype meaning the stuff of inheritance, the payload, and phenotype meaning everything else, the vehicle for the genes.
Only recently did I try to find out why there are two pairs of terms, and what the technical distinction is. I was a little surprised by what I found, which comes from an article at The Embryo Project Encyclopedia titled Wilhelm Johannsen’s Genotype-Phenotype Distinction:
Wilhelm Johannsen first proposed the distinction between genotype and phenotype in the study of heredity while working in Denmark in 1909. The distinction is between the hereditary dispositions of organisms (their genotypes) and the ways in which those dispositions manifest themselves in the physical characteristics of those organisms (their phenotypes). This distinction was an outgrowth of Johannsen’s experiments concerning heritable variation in plants, and it influenced his pure line theory of heredity. While the meaning and significance of the genotype-phenotype distinction has been a topic of debate—among Johannsen’s contemporaries, later biological theorists, and historians of science—many consider the distinction to be one of the conceptual pillars of twentieth century genetics.
Genotype and phenotype are more general terms, referring to “dispositions” rather than cells, and applying as well to plants as animals. The meaning of Weismann’s terms germ and soma, in contrast, were based on what he saw and what was later determined to apply mostly to cellular reproduction in animals.
In describing the results of his experiments with plants, Johannsen referred to the group identity of the organism as its genotype and contrasted this with its phenotype, or the individual qualities of those organisms. The key phrases being group identity versus individual qualities.
Johannsen’s genotype-phenotype distinction has some similarity to August Weismann’s late nineteenth century distinction between the germ and soma, in that both thought that the causal interactions between an organism’s hereditary disposition and its physical characteristics was unidirectional. Although Johannsen acknowledged this affinity with Weismann’s ideas, he was unwilling to engage in what he considered to be unjustified speculations about the material basis of the genotype, as he argued Weismann had done.
It’s important to remember that Johannsen and Weismann did their research and made their fundamental distinctions before anything was known about the molecular-level mechanics, the DNA and biosynthesis within cells. I am perhaps still misunderstanding some subtlety here, but it seems that the more recent biological discoveries of DNA and the general one-way rule inside cells, Crick’s central dogma, have now firmly established the “material basis of the genotype”.
Setting aside the poisonous effects of politicization on science, which I get a whiff of in the wording of the Embryo Project Encyclopedia article on Johannsen, I think much confusion and defensiveness arises when scientists who are attempting to detect patterns and declare “rules” are confronted with a not-so-clean-cut reality. Reality tends to fit some coarse rules but also, due to the interaction of so many moving parts at different scales, turns out to be more complex than scientists at first imagine.
This does not make science or the broader rational pursuit of making sense of reality useless. When farmers who domesticated animals thought of and discussed heredity in terms of bloodlines, they weren’t entirely wrong, they just didn’t appreciate the finer details. Though they were ignorant of those details they were still able to manipulate the rules of reproduction and inheritance as they understood them.
Let’s make sure we understand what the terms genotype and phenotype mean by consulting some other sources. An article titled Evolution 101: Genotype versus Phenotype at berkeley.edu says:
An organism’s genotype is the set of genes that it carries. An organism’s phenotype is all of its observable characteristics—which are influenced both by its genotype and by the environment.
It goes on to provide the example of two cats, one with the usual ears and one with deformed ears, a difference rooted in their genes. As an example of a phenotype being altered by the environment it mentions flamingos, whose pinkness is not encoded in their genotype but results from the food they eat.
Wikipedia’s page on Genotype-phenotype distinction is more of the same, but adds an important aspect of phenotype that we haven’t mentioned yet:
“Genotype” is an organism’s full hereditary information. “Phenotype” is an organism’s actual observed properties, such as morphology, development, or behavior.
Behavior is not something most people think of as concrete in the same sense the physical shape or composition of a protein or cell or body is. “Anti-racists” have constantly and consistently tried to minimize and even deny the heritability of behavior, or personality traits. Though behavioral traits are less tangible, literally more difficult to see than material traits, the heritability of both is a fact that even semitically correct Wikipedia cannot yet erase.
Richard Dawkins coined the term extended phenotype to include all effects a gene has, inside and outside the body. Common examples include things like the dams and dam-building behavior of beavers, and the webs and web-spinning behavior of some spiders. It also includes the civilizations and civilization-building behavior of some races of humans.
Another type of extended phenotype Dawkins identified, which is also evident in humans, is the:
action at a distance of the parasite on its host. A common example is the manipulation of host behaviour by cuckoo chicks, which elicit intensive feeding by the parasitized host birds. These behavioural modifications are not physically associated with the host but influence the expression of its behavioural phenotype.
Dawkins summarizes these ideas in what he terms the Central Theorem of the Extended Phenotype:
An animal’s behaviour tends to maximize the survival of the genes “for” that behaviour, whether or not those genes happen to be in the body of the particular animal performing it.
I’ll bet in their thinking about life, neither Weismann or Johannsen ever dreamed of something so bizarre. Unless maybe they understood something about the jews.
We’ll wrap up this installment with one last pair of terms which are also in line with our germ and soma theme. The terms are haploid and diploid. Diploid cells have two complete sets of DNA, one set from each parent. All soma cells are diploid. Haploid cells, in contrast, have only one complete set of DNA. In animals the germ cells, the gametes (sperm and eggs), are haploid.
Image source: The Cellular Basis of the Immortality of Species.